Metamaterials are a class of material with widely tunable optical properties and show promise for exotic applications such as negative refractive index materials. They are materials with contrasting permittivity (ε) and permeability (μ) arranged in discrete periods below the diffraction limit. The material period should be much smaller than the wavelength (or wavelength range) of interest such that the impingent radiation experiences effective material properties and can be periodic in one-dimension (1D), two-dimensions (2D), or three-dimensions (3D) to offer control over the effective index of refraction anisotropically.
Metamaterials are commonly characterized by their effective index of refraction, n, where n2 = ε.μ. Many examples of metamaterials employ plasmonic metal shapes, using their resonance behaviors to fabricate selective absorbers, emitters, and waveguides.
Due to the nanometer-scale probe size, electron-based excitation, and the recent advent of angle-resolved (AR) and wavelength- and angle-resolved (WAR) modes of operation, cathodoluminescence (CL) proves to be an ideal technique for investigating metamaterials. In the case of the Au-based asymmetric split ring (ASR) resonator metamaterial, CL is useful for observing their emission spectrum and directionality.
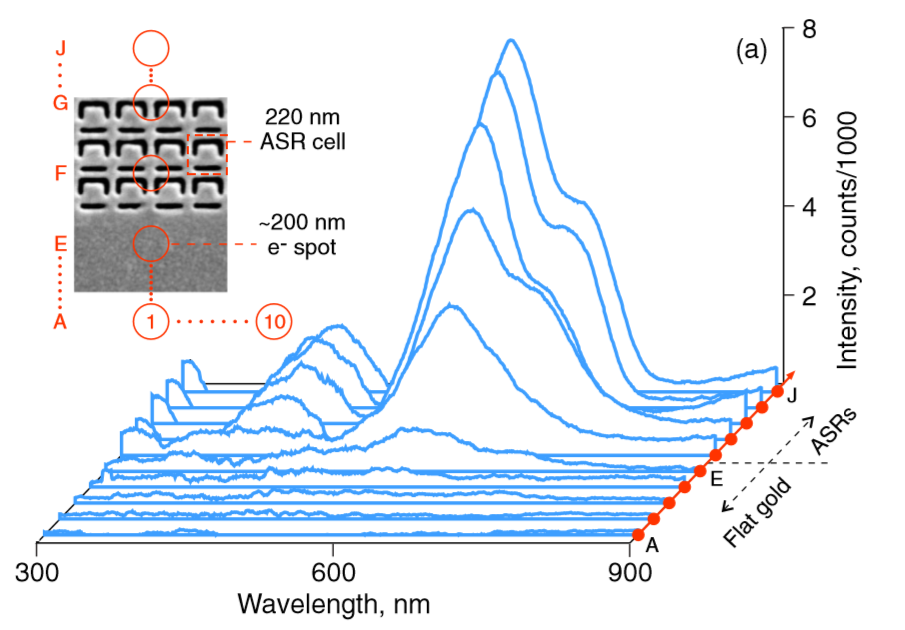
The difference between photonic crystals and metamaterials is period: To have the photonic band gap the atoms and the lattice constant in the former have to be comparable in size with the wavelength. At the same time, the periodicity should be much smaller than the wavelength for the latter.
![]() |
Determining photonic band structure by energy-momentum spectroscopy in an electron microscope |